For centuries, the concept of immortality has fascinated humans, frequently appearing in myths, legends, and science fiction. However, in the microscopic realm of cells, cellular immortality is a startling reality, particularly in cancer cells. These cells masterfully evade the natural limits of normal cell life, leading to unchecked growth and tumor formation. Understanding how cancer cells achieve this immortality is crucial in the fight against cancer.
The Hayflick Limit: A Barrier to Immortality
In the 1960s, scientist Leonard Hayflick reshaped our understanding of cellular aging and cancer with a groundbreaking discovery. He revealed that normal human cells divide only a limited number of times—typically around 40 to 60—before they stop dividing and enter a state known as senescence. This barrier, known as the Hayflick limit, prevents cells from becoming immortal.
To illustrate this, Imagine cells as cars with a limited number of miles they can drive before breaking down. Each time the car is driven, it wears down a bit more until it stops running. Similarly, each time a cell divides, it loses part of its protective caps—telomeres—located at the ends of chromosomes. Telomeres function like the plastic tips on shoelaces, preventing fraying. With each division, the telomeres shorten. Once they become too short, the cell can no longer divide and either enters senescence or undergoes programmed cell death, known as apoptosis.
This process acts as a natural barrier to unlimited cell division, maintaining tissue integrity and preventing unchecked growth that could lead to cancer. However, cancer cells have found ways to bypass this limit, allowing them to divide indefinitely and achieve a form of cellular immortality.
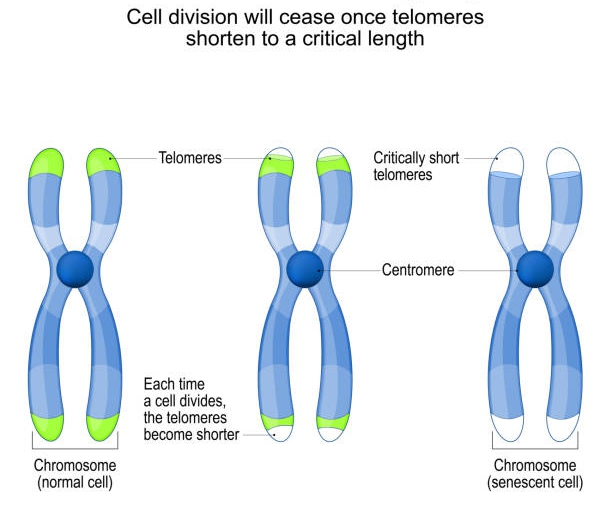
How Cancer Cells Break the Hayflick Limit
Telomere Maintenance: The Key to Endless Division
- Telomerase Activation: Cancer cells reactivate an enzyme called telomerase, which rebuilds telomeres and allows the cells to keep dividing. This reactivation is often driven by mutations in the TERT gene, which encodes the enzyme’s catalytic subunit. By resetting the “cellular clock,” telomerase enables cancer cells to divide endlessly.
- Alternative Lengthening of Telomeres (ALT): Some cancers, such as sarcomas and gliomas, use a different method called ALT to maintain telomeres without relying on telomerase. This alternative pathway provides another route for cancer cells to achieve immortality.
Evading Cellular Senescence: Avoiding the Cell’s Retirement
- Disrupting Growth Signals: Mutations in key genes like p53 and RB—normally responsible for enforcing senescence by halting cell division—allow cancer cells to keep proliferating. By disabling these protective mechanisms, cancer cells continue unchecked growth.
- Oncogenes and Tumor Suppressors: Besides telomere maintenance, oncogenes (which promote cell division) and tumor suppressor genes (which inhibit it) play a crucial role in cancer cell immortality. Activating oncogenes and inactivating tumor suppressors enable cancer cells to evade senescence and continue dividing.
Blocking Apoptosis: Resisting Cell Death
Normally, severely damaged cells self-destruct. However, cancer cells produce proteins that block this process, allowing them to survive and keep dividing despite the damage.
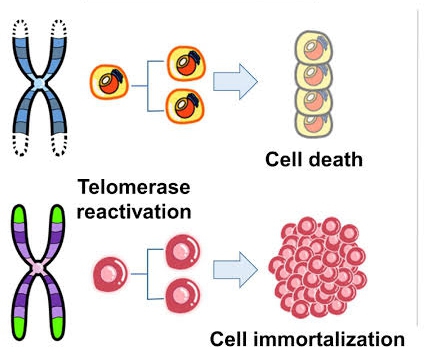
Therapeutic Implications: From Immortality to Vulnerability
Cancer’s ability to surpass the Hayflick limit and achieve immortality presents a paradox—it poses significant dangers while also offering potential opportunities for treatment. While this ability fuels the relentless growth of malignant tumors, it also results in genetic instability. This instability enables cancer cells to evolve and adapt more rapidly, but it also creates vulnerabilities that treatments can exploit. The very trait that makes cancer so dangerous could become its greatest weakness.
Researchers have used these mechanisms to develop therapies that specifically target the pathways enabling cancer cells to divide indefinitely.
Telomerase Inhibitors
- Direct Inhibitors: Drugs like imetelstat (Rytelo) directly inhibit telomerase activity and have been approved for treating low- to intermediate-risk myelodysplastic syndrome (MDS) with transfusion anemia. Research is also ongoing to evaluate their efficacy in solid tumors.
- TERT Vaccines: Vaccines targeting the telomerase reverse transcriptase enzyme(TERT) aim to stimulate the immune system to attack cancer cells expressing TERT.
Targeting the ALT Pathway
- ALT-Specific Inhibitors: Researchers are working on developing inhibitors of the ALT pathway, which could be effective against ALT-positive tumors.
- PML Body Disruption: Compounds that disrupt ALT-associated PML bodies—nuclear structures involved in the ALT mechanism—could interfere with telomere maintenance in cancer cells.
Restoring Senescence Pathways
- P53 Reactivation: Small molecules such as eprenetapopt (APR-246) are being explored in clinical trials for their potential to restore mutant p53 function.
- CDK4/6 Inhibitors: Drugs like palbociclib (Ibrance) inhibit cyclin-dependent kinases 4 and 6, reactivating the retinoblastoma (Rb) pathway and enforcing cell cycle arrest.
Inducing Apoptosis
- BH3 Mimetics: Venetoclax and similar drugs mimic pro-apoptotic proteins, inducing apoptosis in cancer cells.
- Death Receptor Agonists: Researchers are investigating agonists targeting death receptors to trigger apoptosis in resistant cancer cells.
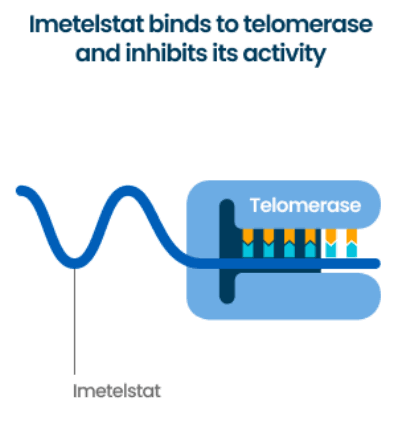
Challenges and Future Directions
The immortal nature of cancer cells presents several unique challenges in treatment:
- Therapeutic Targeting of Telomerase
Targeting telomerase shows promise, but challenges remain. A primary concern is the potential for side effects, as telomerase is also active in certain normal cells like stem and germ cells. Selectively targeting cancer cells without harming normal tissues is critical for the success of telomerase inhibitors.
- Overcoming Resistance Mechanisms
Cancer cells can develop resistance to telomerase inhibitors and other therapies targeting telomere maintenance. Understanding these resistance mechanisms and developing combination therapies are essential research areas. Combining telomerase inhibitors with chemotherapy, radiation, or immunotherapy may enhance effectiveness and prevent resistance.
- Tailoring Treatments to Individual Patients
Cancer is a heterogeneous disease, meaning different patients—even with the same cancer type—may have unique underlying mechanisms driving their disease. Personalized medicine, which tailors treatments to a patient’s specific genetic and molecular cancer characteristics, is vital in effectively targeting cancer cell immortality.
Conclusion: Challenging the Eternal Life of Cancer Cells
Cancer’s ability to exceed the Hayflick limit demonstrates its resilience and adaptability. By overcoming natural barriers to cell division, cancer cells achieve a form of immortality that fuels their relentless growth. However, this same immortality represents a significant vulnerability. Targeting the mechanisms that allow cancer cells to maintain their telomeres and evade senescence provides an opportunity to develop more effective cancer therapies.
The fight against cancer is far from over, but with each discovery, we come closer to finding ways to turn cancer’s immortality into a weakness. The ultimate goal is to extend life without sacrificing quality, ensuring that cancer’s pursuit of immortality no longer threatens human life. As we continue unraveling cancer biology’s mysteries, we move closer to a future where cancer’s immortality leads to innovative, life-saving treatments.
Further Readings
Artandi, S. E., & DePinho, R. A. (2010). Telomeres and telomerase in cancer.
Shay, J. W., & Wright, W. E. (2019). Telomeres and telomerase: Three decades of progress.
Zhang JM, Zou L. Alternative lengthening of telomeres: from molecular mechanisms to therapeutic outlooks.
O’Sullivan, R. J., & Karlseder, J. (2010). Telomeres: Protecting chromosomes against genome instability.
Hanahan, D., & Weinberg, R. A. (2011). Hallmarks of cancer: The next generation.